|
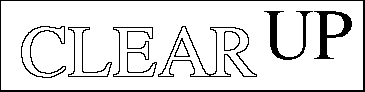 |
|
March
1991
AT&T Bell Labs 600 Mountain Ave.
Murray Hill N.J. 07974
Copyright © 1991 AT&T All Rights Reserved |
Why
is the sky blue? |
This famous question was first answered correctly
by Lord Rayleigh in 1881. He recognized that the air is full of
particles (e.g. dust, gas molecules, etc.) which scatter light.
The kind of scattering depends on the particle size. If the particles
are much larger than the size (wavelength) of light (about one millionth
of a meter, which is about 1/25th the diameter of a human hair),
the light is scattered uniformly in all directions independent of
the wavelength.This is why clouds, which consist of water droplets,
are white and fluffy.
If the particles are about the size of the wavelength of
light or smaller, the amount of scattering depends on the wavelength
of the light. (And thus the color. Blue light is "smaller"
than red, by about a factor of 1/2). If the wavelength is a little
longer than the dust particle is wide, not too much scattering occurs.
A good analogy is a wave at the beach hitting a piling. A gentle,
long period wave is barely disturbed, and just raises and lowers
the water level at the piling. However, a wave about the size of
the piling is scattered sideways.
Thus,
the effect of dust is to let the long wavelength (RED) light pass
through, while scattering to the sides the short wavelength (BLUE).
In this way dust takes the white light from the sun and reorganizes
it by wavelength. The background color of the sky comes from scattered
light, and is blue, while the image of the sun comes from
direct light, and is yellow-red.
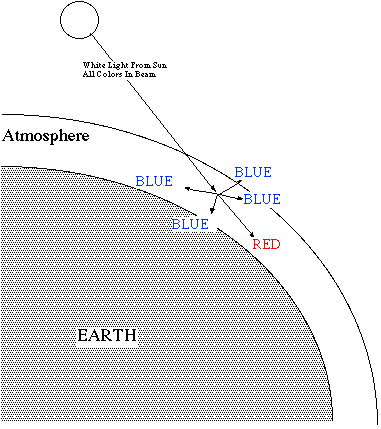 |
If the number of scatters is very
large, the sun will appear blood red. This usually occurs on a dusty
day, after volcanic eruptions (the sun set red around the world for
a year after the great explosion of Krakatoa in 1883), or at sunrise
or sunset, when the light from the sun is almost tangent to the ground
and so goes through a longer length of the atmosphere. An excellent
resource on all aspects of color and optical illusions is the charming
book "The Nature of Light and Color In The Open Air", by
Minnaert, and there is a clear discussion by Jackson in his "Classical
Electrodynamics" book. A good resource for experiments is "Clouds
In A Glass of Beer- Simple Experiments in Atmospheric Physics"
by C.F. Bohren.
The traditional demonstration illustrating the effect of
"Rayleigh Scattering" is to shine a 35 mm projector through
the side of a rectangular fish tank filled with water. Milk is slowly
mixed into the water. As the milk is added, the circle of light
on the wall will turn red, and the tank of water, blue. |
What
is the Difference Between A Glass, a Solid and a Liquid? |
Glass
is sometimes referred to as being a "liquid". This is
a little bit confusing, in that glasses usually appear to be quite
rigid just like any other solid material. In fact, almost all glasses
can quite accurately be described as being a liquid which has been
cooled so far below its melting point that it is extremely viscous
and thus has the mechanical properties of a solid. The reason glasses
are described as being "liquids" is to distinguish them
from other solids that are "crystalline" in nature. In
a crystal every atom is precisely arranged. Each atom is a certain
distance from its neighbors, and the bonds between atoms always
have the same angles with other bonds. In a liquid the molecules
that make up the liquid are constantly moving with respect to one
another, so that if you wait a fraction of a second all of the molecules
will have rearranged themselves. A glass is like a snapshot in time
of a liquid. The distances between atoms are not always the same,
nor are the angles between them. But, like a crystal, the position
of the atoms rarely change over human time scales.
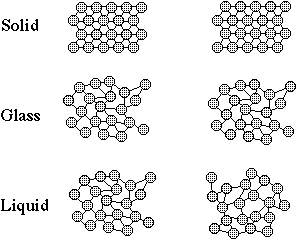 |
Here is a simple experiment using freezing to illustrate
the distinction between a crystalline phase and a glass. Put some
water and some corn syrup into the freezer. As the water gets colder,
needles of ice form and grow, eventually taking over the whole volume.There
is always a sharp boundary between the ice and water, because ice
(a crystal) and water (a liquid) have different structures. The
corn syrup just gets thicker and thicker until it gets so thick
that it won't noticeably flow. At no time is there a sharp boundary
between fluid and solid phases, because the syrup has not gone through
a change of structure. It remains "glassy" at all times.
In a glassy material like a window pane (made up in large
part by silicon and oxygen atoms) the atoms do not appreciably rearrange
themselves, unless you are willing to wait millions of years. A
silicon atom will remain firmly attached to a certain oxygen atom
which will in turn be firmly attached to another silicon atom.The
only disorder is that which was described earlier, in which bond
lengths and angles are somewhat varied from atom to atom in the
glass.
There is an old story that "proves" glass
is a liquid because it was "observed" to flow over the
centuries. In colonial houses, the window panes are often found
to be thicker on the bottom than the top. People then erroneously
drew the conclusion the glass, being a liquid, had sagged under
the pull of gravity. In fact, old glass is thicker on one edge when
first made, and does not change shape. In the past, glass sheet
was made by blowing a ball of hot glass into a hollow globe, and
then slowly forming into a cylinder. The cylinder was cut apart
and laid out flat into a sheet. The resulting window panes have
small air bubbles, wrinkled, picked up dirt when cooling, and tended
to have one thick-end. The window installer would systematically
place the thick-end down (where are today's craftsmen?), thus setting
off centuries of idle, and ultimately, wrong-headed speculation
on the behavior of glassy material. If glass flowed by an eight of an inch over two centuries, then scratches would seal-over in days- and that never happens.... See this article from the Corning Glass Museum for more details. |
Are
Zebra Mussels a Problem in Europe? |
Zebra mussels were first described in rivers emptying into the Caspian
Sea in the 18th. century. They were introduced into European waters
roughly 150 years ago, where they underwent a population explosion
comparable to the one now occurring in this country. The population
levels of the zebra mussel in European waters have fallen due to
adjustments in behavior and population levels of their predators,
e.g., fish, birds and crustaceans. Zebra mussels are now integrated
into the food chain in European waters but they are still considered
a pest species and still cause significant fouling of pipes and
boats in European waters. In the U.S., the mussels have recently
invaded the Great Lakes. They form dense colonies of more than 30,000
mussels per square meter. Since each mussel can filter one liter
of water in 24 hours, in some locations the volume of the lake is
completely filtered each day by it's bivalve invaders! Needless
to say, the ecological impact is enormous.
An excellent source of further information on all aspects
of zebra mussel ecology and economics is The Zebra Mussel Clearing
House at the State University of New York at Brockport. The co-ordinator
is Susan Moore (716 395-2516). They have a great deal of free literature,
including a 10 page fact sheet, 3 complimentary issues of a newsletter,
photocopies of published papers, a bibliography($2.00) and specialists
to answer technical questions. |
Why
do we divide the day into 24 hours, an hour into 60 minutes, etc.? |
The 24 hour day and 60 minute hour originated with the Babylonians,
who based their calendar on the earlier Sumerian and, perhaps, Egyptian
calendars. The Encyclopedia Britannica has a number of good articles
on this subject, and there is a wonderful chapter by Daniel J. Boorstin
in "The Discoverers". As Boorstin explains, the history
of timekeeping is itself lost in time.
The origin of a counting system based on 60 is related to
astronomical cycles in the earth-moon-sun system. The earth takes
about 360 days to orbit the sun, and over the course of a year the
moon goes through about 12 cycles. Our life today is not very well
keyed to these astronomical periods, but following and understanding
the flow of the seasons was a great deal more important in earlier
societies.
The Sumerians were probably the first to develop a calendar
based entirely on the phases of the moon. The Babylonians refined
this idea to form a calendar of which several details survive in
our"modern" calendar.-A Babylonian month began on the
first day of a new moon.-There are slightly more than 12 Babylonian
months in a year (about 12.37 to be more precise). [Every two or
three years, the royal astronomers would insert an extra full month
into the calendar...so that the calendar based on the moon's cycles
would stay in better sync with the year]-From the fact that there
are about 12 lunar months in a solar year, the Babylonians decided
that the number 12 is the logical number by which time should be
further divided.-From about 300BC, the Babylonians used a hemispherical
sundial-type device to keep time during the day. The shadow cast
by a thin rod would travel past 12 equally spaced marks on the inside
of a hemisphere. The time it took for the shadow to pass from one
mark to the next was ONE HOUR. Thus there were 12 hours during daylight.
The night time was also divided into 12 parts, as timed most likely
by water clocks...the other type of Babylonian clock. (Note that
this Babylonian system yields hours which are shorter during winter.when
the days are shorter.and longer in summer. As a result, in Babylon,
in modern-day Iraq, the daylight hours in mid-winter were only 2/3
as long as the mid-winter night time hours with the opposite situation
occurring in mid-summer.
The invention of accurate mechanical clocks at the end of
the 13th century finally made the variable-length hour less useful
than the modern hour of unchanging duration.-The Babylonians divided
the hour into 60 minutes (60 = 5 x 12....that preferred number 12
appears again). Additional Questions:
-
2000 years ago, how would you determine if the year was 365 or
364 days long?
-
The day was not always 24 hours long. Half a billion years ago,
a day (one complete rotation of the earth) lasted about 20 of
our present hours. Since the day is set by rotation of the earth,
and angular momentum must be conserved, how did the day change
in length?
-
If the year were 100 days long, with 10 months to the year, we
might have adopted the decimal system a little earlier (especially
with the attraction of 10 fingers and 10 toes to count on). But,
what effect would a three times longer day have on our climate,
tides, etc?
|
Fruit
Flies and Power Lines |
A student called in asking how to set up a 110V circuit to
mimic the electric and magnetic fields around a high voltage line.
They are working on a science fair project, and wanted to
see if these fields might effect the growth and death of a colony
of fruit flies. Now, setting up a solenoid and a capacitor
plate to give similar field distributions is straightforward,
but designing an experiment to detect possible biological
effects is not. It is a good illustration of how hard experiments
are to perform when the effects are small and the experimental conditions
are out of your direct control.
The first problem you encounter is the same one epidemiologists
must overcome in studies on humans. Although the fields right
next to a power line can be high, they drop off rapidly with distance.
Measurements show the distribution of field strengths in homes close
to power lines overlaps the distribution of electric and magnetic
fields in homes miles away from any power line. Thus, simply trying
to correlate the address of people's houses with the cancer rate
is likely to give spurious results. People near the line who
use gas heat, mechanical clocks, and in general very little
electricity could get a lower field exposure than those in an electrically
heated apartment building miles away. Similarly, the students could
set up their experiment in the basement, with the "control"
population of flies near the main power feed to the house. These
fields might be greater than those in their solenoid encased "exposed"
population. Careful experiments (including monitors worn by people
to measure their actual exposure) will be needed to settle
the issue in human populations. The cover story in April's issue
of Radio Electronics is on magnetic fields and "how-to-build"
simple devices to measure your exposure (however, their choice
of references is a little biased in favor of possible links to cancer
).
The second problem you'll encounter is a matter of
statistics. No reputable investigation has found that power lines
cause big effects; your fruit flies won't just drop dead when
you put the wires nearby. After all, people don't drop dead when
they walk under power lines either. For people, the argument
is about 1% or so (possible) extra deaths from cancer over
a period of years. So, if we assume that fruit flies behave like
people (not always a good assumption), you should expect that most
flies will live a normal fly lifespan, and die at about the same
rate whether the field is on or off. Now let's think about some
results we might get. Assume 12 flies died in bottle A, and 11 in
bottle B. Does this mean that there is a real difference between
the two bottles? Not necessarily. One of the flies could have been
less healthy in bottle A, perhaps. You might as easily find two
flies which lived longer in a bottle near the power line.
Does this mean we should recommend power lines as a health
tonic? The problem is, you have to deal with large enough
numbers so that individual, random differences between the bottles
are less important. If you made the experiment 10 times bigger,
so that 120 flies died in bottle A and 110 in B, you'd have a better
result. One unhealthy fly can no longer tip the scales - you
have to have 10 sick flies in the same bottle. If you make
the experiment even bigger so that A had 1200 and B had 1100 - then
you'd really have something.The odds of getting 100 extra sick flies
into bottle A would be pretty small. You have to have enough
flies so that even a small difference between the two groups
is still much more than the random fluctuations in the death
rate. So, if you want to look for a small difference, you're going
to have to count a lot of fruit flies (dead and alive).
A lot also depends on how the electromagnetic field
affects the death rate. If fruit flies never die before one
month of age, and with exposure to the field 1% start dying at one
week, a field induced effect could be claimed. However, it
is more likely flies die all the time, and we are forced to
look for an increase in the average death rate by 1%. Lets say 10%
of the flies normally die in the first month, so a 1% increase in
the death rate corresponds to a new death rate of 11%. If we had
1,000 flies in the bottle, we need to tell the difference
between 100 dead flies and 110 dead flies at the end of a
month. This sounds simple (but tedious) enough, except that the
10% figure is an average number. You expect in some months more
flies would die than average, and some month less (that's what average
means). In many cases, the square root of the expected number
is a good measure of the fluctuations. In this case, the square
root of 100 is 10, so you might expect 100+-10 to die even
without the field. This means you cannot tell if the 110 represents
additional deaths due to the field, or just a random fluctuation
above the average. Here is how the confidence level increases as
you increase the number of flies in the bottle.
Number
of Flies |
10%
Deaths (with no field) |
11%
Deaths (with a field) |
Deaths
Due to Field |
Deaths
due to random statistics
|
100 |
10
|
11
|
1
|
3
|
1,000
|
100
|
110
|
10
|
10
|
10,000
|
1000
|
1,100
|
100
|
33
|
100,000
|
10,000
|
1,000
|
1,000
|
100
|
|
You can see that it becomes a very hard experiment if you
want really accurate answers, or if you want to see a small
difference. (With 100,000 flies, the difference in death rate with
and without the field is 1,000 flies, compared to random fluctuations
of 100 flies. This would be considered a "statistically
significant" experiment). That's exactly why there is
still argument about the effect of power lines on people.
You'd have to keep track of a LOT of people to be sure that a difference
is real, and not just chance.
You also have to be sure the flies ate the same food, got
the same lighting, and so on. With people you have to compensate
for diet, smoking, workplace related disease, etc. Otherwise, when
looking for small effects, uncontrolled (sometimes called "systematic")
errors can swamp your results. Ultimately, science is done
by people in a real and challenging environment. Mistakes
happen, even to people with the best training and intentions.
Over the long term, however, we like to believe the best and most
accurate results are reproduced, survive, and are finally taught
to our children and the public
In
the next newsletter:
- What
happens when electrons scatter off electrons?
How fast does the earth cool?
What are imaginary numbers good for?
|
NOTE:
THIS ADDRESS AND PHONE NUMBER IS NO LONGER ACTIVE! (as of 1993)
|
If you have any comments on this newsletter, call
1-800-CLEARUP or write
- CLEARUP
c/o AT&T Bell Labs
600 Mountain Ave.
P.O. Box 636
Murray Hill, N.J. 07974
|
|
|